Major upgrades and a new, state-of-the-art facility will support the nuclear weapons stockpile for decades to come.
March 24, 2025
During the last week of December, Los Alamos National Laboratory ceases all non-essential work for its annual winter closure. Among other things, the closure marks the end of a five-month “run cycle” at Los Alamos Neutron Science Center (LANSCE)—a kilometer-long particle accelerator that Los Alamos has operated for more than half a century. When the Laboratory reopens after the New Year, workers spend months completing extensive maintenance at LANSCE, readying the facility for its next run cycle when the accelerator will once again generate high-energy protons that are used for everything from creating cancer-treating medical isotopes to imaging small-scale explosions.
In late 2023, however, LANSCE—which has suffered increasing downtime as it has aged—faced a backlog of critical experimental work. This backlog meant that LANSCE would need to run until February 2024, including during the winter closure. On Christmas Day, Eric Brown, who directs LANSCE’s user facility, made a trip to LANSCE with food and cookies for staff who were there to keep the accelerator running. “I was expecting that they’d be frustrated to be at work during the holidays,” Brown says. “But they were having a great time, working together and enjoying the collegial atmosphere.”
That dedication is characteristic of those who work with the Laboratory’s accelerators, Brown says, and arises out of the collaboration that working with such complex machines necessitates. And yet, the need to alter LANSCE’s run-cycle schedule—which was caused in part by the failure of crucial accelerator components—reflects problems related to the facility’s age (the accelerator celebrated its 50th birthday in 2022).
LANSCE isn’t Los Alamos’ only major accelerator facility. The Laboratory is also home to the Dual-Axis Radiographic Hydrodynamic Test (DARHT) facility, which is used to conduct crucial experiments related to nuclear weapon components. Like LANSCE, DARHT is aging: The accelerator has been in use for around 25 years—long enough that many of its parts are, or will soon be, in need of replacement.
In addition to the challenges that go along with age, LANSCE and DARHT are being called upon to support more experiments than ever. Shea Mosby, of the Laboratory’s Accelerator Strategy Office (ASO), says that each of LANSCE’s five experimental areas—which use LANSCE’s accelerator beam to conduct different kinds of research—are overburdened.
“Right now, LANSCE’s user program is oversubscribed by a factor of between two and three,” Mosby says. “And, at the same time, our reliability has been decreasing.”
The ages of LANSCE and DARHT and the need for increased experimental throughput to support national security objectives are driving major initiatives to modernize the Laboratory’s two flagship accelerators. In the next decade, the LANSCE modernization project will completely replace the front end of the accelerator, ensuring that LANSCE can carry out crucial national security research until 2050 or beyond. Meanwhile, the DARHT Capability Expansion project is refurbishing DARHT and preparing the accelerator for new types of experiments.
Los Alamos’ accelerator expertise is why the Laboratory is also leading the development of a third major accelerator, the Advanced Sources and Detectors/Scorpius project, at the Nevada National Security Sites in southern Nevada.
“Los Alamos is NNSA’s accelerator laboratory,” says program director and ASO leader John Tapia, referring to the National Nuclear Security Administration, which oversees the laboratories, production plants, and national security sites that collectively support the nation’s nuclear stockpile. “The Laboratory operates LANSCE and DARHT, and we’re leading the efforts to construct Scorpius. These three accelerators, plus Lawrence Livermore National Laboratory’s Flash X Ray induction linear accelerator, are the large-scale accelerators that make up the heart of NNSA’s accelerator complex.”
In 2023, the Laboratory’s deputy director for Weapons and deputy director for Science, Technology, and Engineering jointly created the ASO to steward the Laboratory’s accelerators and help ensure that new initiatives and technologies are brought to bear on facilities like LANSCE and DARHT. Tasked with developing an integrated accelerator strategy (which will be published this year) to ensure that these and other tools meet critical mission needs into the future, the ASO is helping unify Los Alamos’ accelerator capabilities.
The ASO also indirectly supports the Scorpius project, which has recently met important milestones on its way to completion. Scorpius will build on the Laboratory’s decades of accelerator experience, allowing researchers to carry out important experiments with plutonium. In the 2030s, when Scorpius comes online and the upgrades to LANSCE and DARHT are completed, the Laboratory—and the nuclear security enterprise—will have a comprehensive, complementary suite of tools to support the nation’s stockpile for decades to come.
“As we get further and further away from historical underground nuclear testing, we’re going to need the ability to do experiments that will help us answer important questions about our weapons,” Tapia says. “We’re working to understand what’s necessary to sustain or modernize these accelerators so that we can future-proof them.”
LANSCE
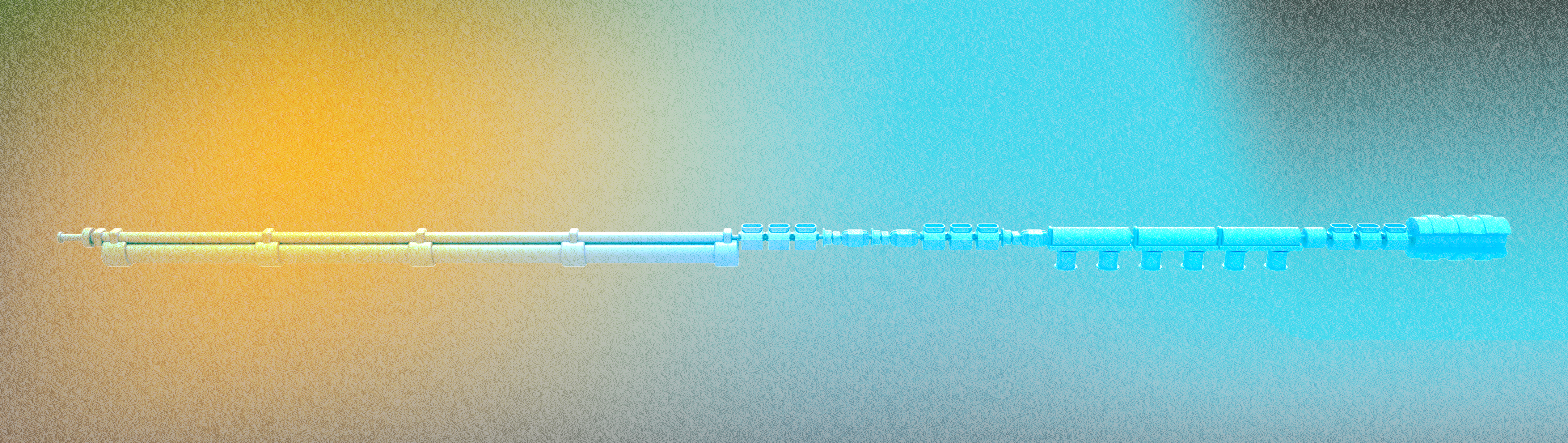
In 2018, LANSCE’s operators discovered a problem: a 12-inch crack in one of the facility’s four accelerating tanks.
The accelerating tanks are part of LANSCE’s “front end”—the first part of the accelerator, where protons are generated and accelerated up to 100 megaelectron volts (MeV). As the protons travel along the accelerator, they are subjected to electric fields that accelerate them up to 800 MeV. By that point, the protons are moving at 84 percent of the speed of light—fast enough to travel around the Earth almost 20 times per second (or to reach the Sun in a little less than 10 minutes).
Until the crack in the accelerating tank was repaired, high currents wouldn’t flow through the tank, and LANSCE couldn’t operate at full power. Fortunately, the crack was discovered during one of LANSCE’s annual scheduled maintenance periods, and a welder was able to enter the tank and repair the crack. If the crack had been elsewhere—say, in the fourth accelerating tank, which is too radioactive for workers to enter—the damage would have been irreparable, and LANSCE could have gone offline indefinitely.
Although a worst-case outcome was averted, the incident underscored how LANSCE’s aging components can fail in ways that put the whole facility at risk. LANSCE comprises thousands of parts whose failure could take the accelerator offline, and such failures are why LANSCE’s beam only operates between 60 and 85 percent of the time that it is expected to.
“There are a few accelerators in the nation that are slightly older than LANSCE, but they have all had one, two, or more major modernization programs. We’re still waiting on our first,” Brown says. “We have to turn away key mission deliverables with some regularity because we just don’t have the ability to deliver on them.”
LANSCE is a linear accelerator, meaning that it accelerates particles—in this case, protons—in a straight line (rather than in a circle, as other kinds of accelerators do). Originally named the Los Alamos Meson Physics Facility, LANSCE was commissioned in 1972 to support research into some of the universe’s most basic particles. Over the decades, LANSCE has proven a versatile tool, and new experimental areas installed along the “beam line”—the path that protons travel along the accelerator—have enabled LANSCE to support a broad range of fundamental and applied research endeavors. By accelerating protons into different kinds of targets, it is possible to produce everything from medical isotopes that are key to cancer treatments (at the Isotope Production Facility) to neutrons traveling at incredibly slow speeds that elucidate the properties of fundamental particles (at the Ultracold Neutron Source).
From the beginning, LANSCE has been an “open” facility that can be used by researchers from universities and other non-Laboratory institutions. In recent years, LANSCE has played an increasingly important role in supporting nuclear weapons–related research and development. Unlike DARHT and Scorpius, which test how weapon components work together in a system, LANSCE is suited for investigating, with a high degree of precision, individual components. These “focused” weapon experiments complement the other facilities’ “integrated” experiments, which test (or, in Scorpius’ case, will test) mockup systems.
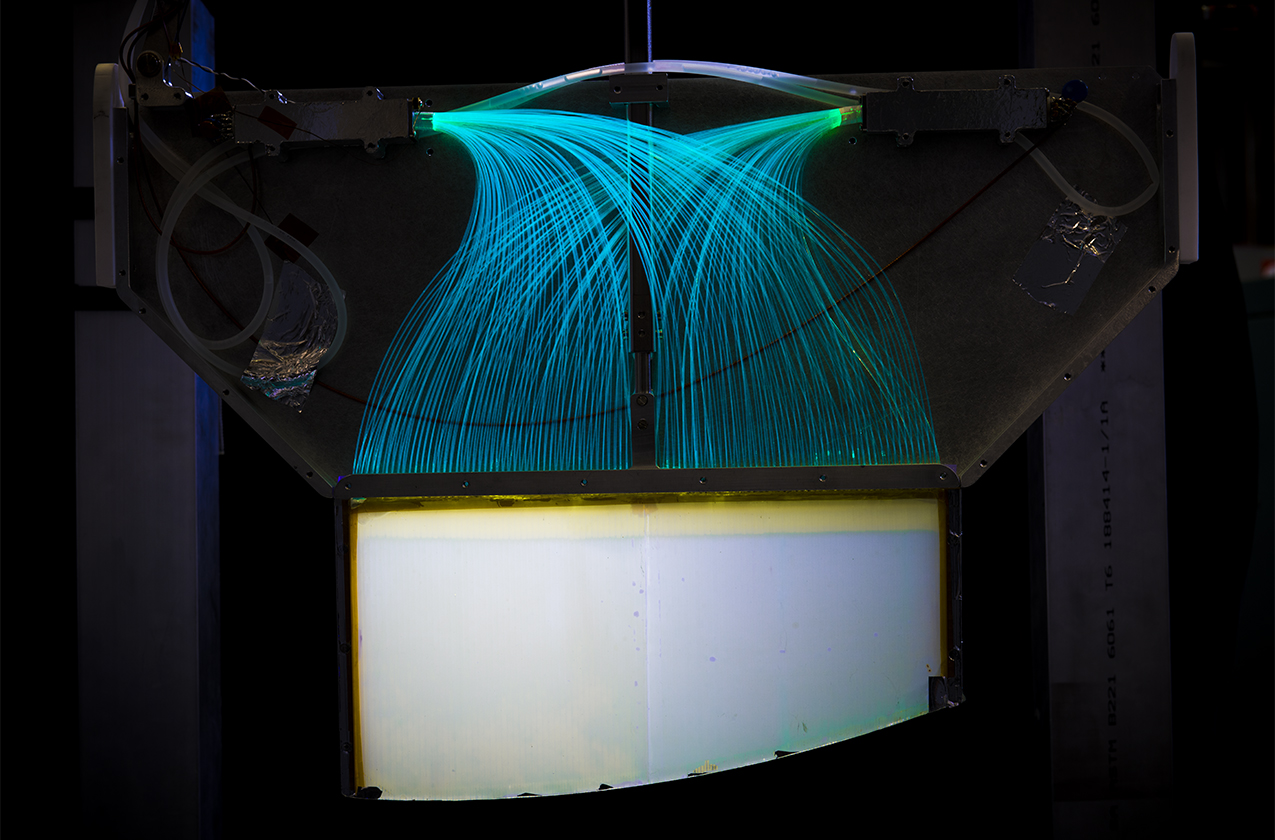
“An integrated experiment frequently tells you what to worry about, but it tends to provide less detail as to why,” Mosby says. “LANSCE comes into play when you have a complicated problem that you need to understand with relevant, underlying science.”
Among the five experimental areas at LANSCE, the Proton Radiography facility, or pRad, has played an increasingly important role in weapons research and development. Using proton radiography—an imaging technique that was developed at Los Alamos in the 1990s—it is possible to make short movies (consisting of around 20 frames) of materials as they are detonated with high explosives.
In 2025, for the first time in two decades, a series of experiments involving plutonium will be undertaken at pRad. By detonating high explosives around very small amounts of plutonium, researchers expect to gain important insights into the metal composing the pits that are the heart of modern nuclear weapons. Data from these experiments will complement the insights to be gained from experiments at Scorpius, which will test larger quantities of plutonium.
Neither pRad nor LANSCE’s other experimental areas will be usable if the facility’s front end fails, however. “Given the age of the facility and the critical downtime issues that we might encounter, the decision was made by NNSA to start a major project, which is the LANSCE Accelerator Modernization Project, or LAMP,” says Greg Dale, LAMP’s director. “LAMP’s goal is to fix key risks and to modernize the front part of the facility.”
LAMP, a billion-dollar project that aims to keep LANSCE operational until 2050 or beyond, involves replacing every part of the front end of the accelerator. This section is responsible for producing protons and providing them with a first burst of acceleration on their journey from one end of LANSCE to the other.
A key part of LAMP will be replacing LANSCE’s two Cockroft-Walton generators. These generators turn a relatively small amount of electrical current into a much higher current, which is used to give protons their initial acceleration. LANSCE’s Cockroft-Walton generators are original to the accelerator and based on designs created in the 1930s. “So, you’re running a vital accelerator with 50-year-old hardware, but the technology is even older than that,” Tapia says. “There aren’t many people in the world who know how to disassemble or replace equipment like this when it fails.”
The Cockroft-Walton generators will be replaced with radio-frequency quadrupoles (RFQs). RFQs, which were developed at Los Alamos in the late 1970s, use alternating electric fields to accelerate protons while simultaneously compressing the protons into groups, or “bunches,” that travel down the accelerator. RFQs are simpler and more reliable than Cockroft-Walton generators, and they are more efficient, too. RFQs have long since replaced Cockroft-Walton generators at accelerator facilities around the world (LANSCE is the only major accelerator facility in the United States that still relies on the latter).
As a part of LAMP, researchers are using a demonstration RFQ to ensure that these components are compatible with LANSCE’s unique requirements, so that the upgrade, when it takes place, goes as smoothly as possible. LAMP recently received approval from NNSA, formally establishing the project and inaugurating the process of conceptual planning and design. The upgrade itself is expected to take place in 2030, and the project team hopes to have it completed in around 18 months. “That’s quite an aggressive timeline, but we need to provide beam to users. That’s why we’re here,” Dale says.
The ASO is working to develop longer-term plans for upgrades to the three experimental areas that are most relevant to national security research (the Lujan Neutron Scattering Center, pRad, and the Weapons Neutron Research Facility). Such upgrades will help ensure that the front-end upgrades completed under LAMP yield high-quality data in support of NNSA’s mission. LAMP is “the first major step” toward modernizing LANSCE, Brown says.
Mosby notes that ensuring LANSCE’s reliability also helps attract talented young researchers to the Laboratory. Students often come to LANSCE to conduct unclassified, fundamental research, returning after graduation to support national security–related work. “Beyond direct programmatic deliverables, LANSCE helps ensure that the Laboratory will have the people it’s going to need through 2050 and beyond,” Mosby says.
DARHT
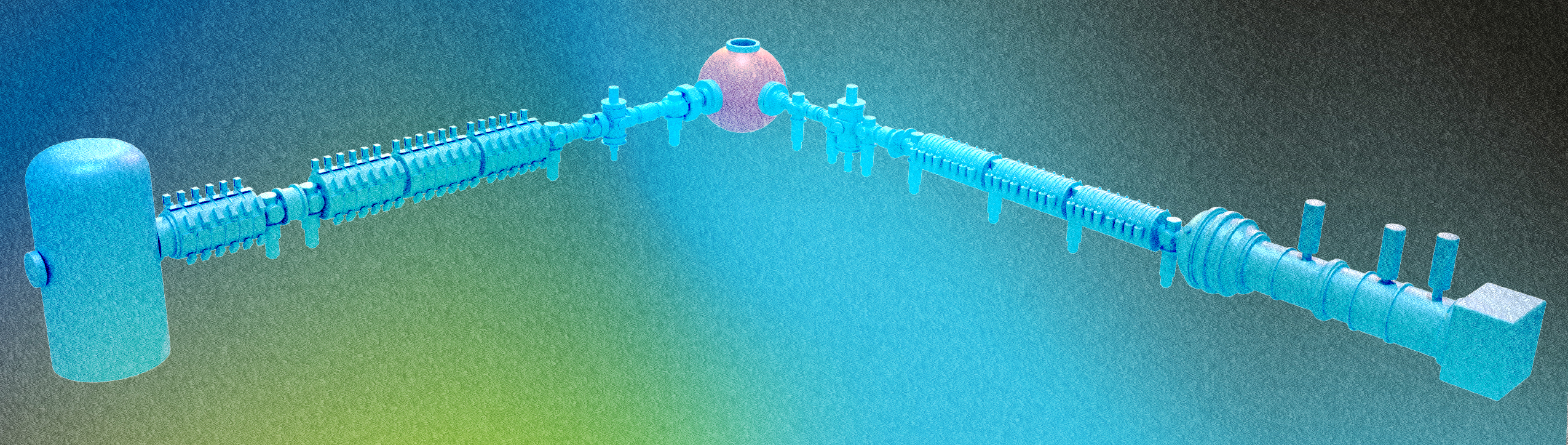
In some respects, the primary way DARHT is used—for hydrotests—resembles an enormous dental x-ray machine. When a dentist presses “Start” on an x-ray machine, a stream of electrons accelerates down a tube and into a heavy-metal target, which is usually made of tungsten. As the electrons pass near the nucleus of the target’s atoms, they emit x-rays, which are directed through the object to be x-rayed and into a detector. The detector—which is a kind of camera—translates the x-rays it receives into an image (cavities and all).
Like a dental x-ray machine, DARHT converts beams of electrons into x-rays by sending the electrons into heavy-metal targets. But while dental x-rays are used to image bone and gum tissue, DARHT’s twin x-ray machines, which are arranged in an L shape around the “firing site,” capture radiographs of mockup nuclear weapon assemblies that implode at speeds greater than 10,000 miles per hour.
In a real weapon, some of these components would be made with plutonium. In DARHT hydrotests, however, the components are made with a surrogate, non-plutonium metal. The implosion rapidly heats the surrogate to temperatures high enough to cause it to flow like water—which is why many DARHT tests are called hydrodynamic tests, or “hydrotests” for short.
Unlike a dental x-ray, which captures a static object, in imaging the implosion of a mock weapon assembly, DARHT must capture a process that takes place in a few millionths of a second. DARHT is also far more powerful than any x-ray machine used for medical purposes (in fact, DARHT is the most powerful x-ray imaging machine in the world).
DARHT’s second axis includes a “kicker”—a device that chops the beam of electrons into four separate pulses. Each of these pulses can be used to create a separate image, and by combining the single image from axis one with each of the four images from axis two, researchers can create 3D mockups of an experiment. Data from DARHT hydrotests can then be compared with computer simulations that predict weapons’ performance.
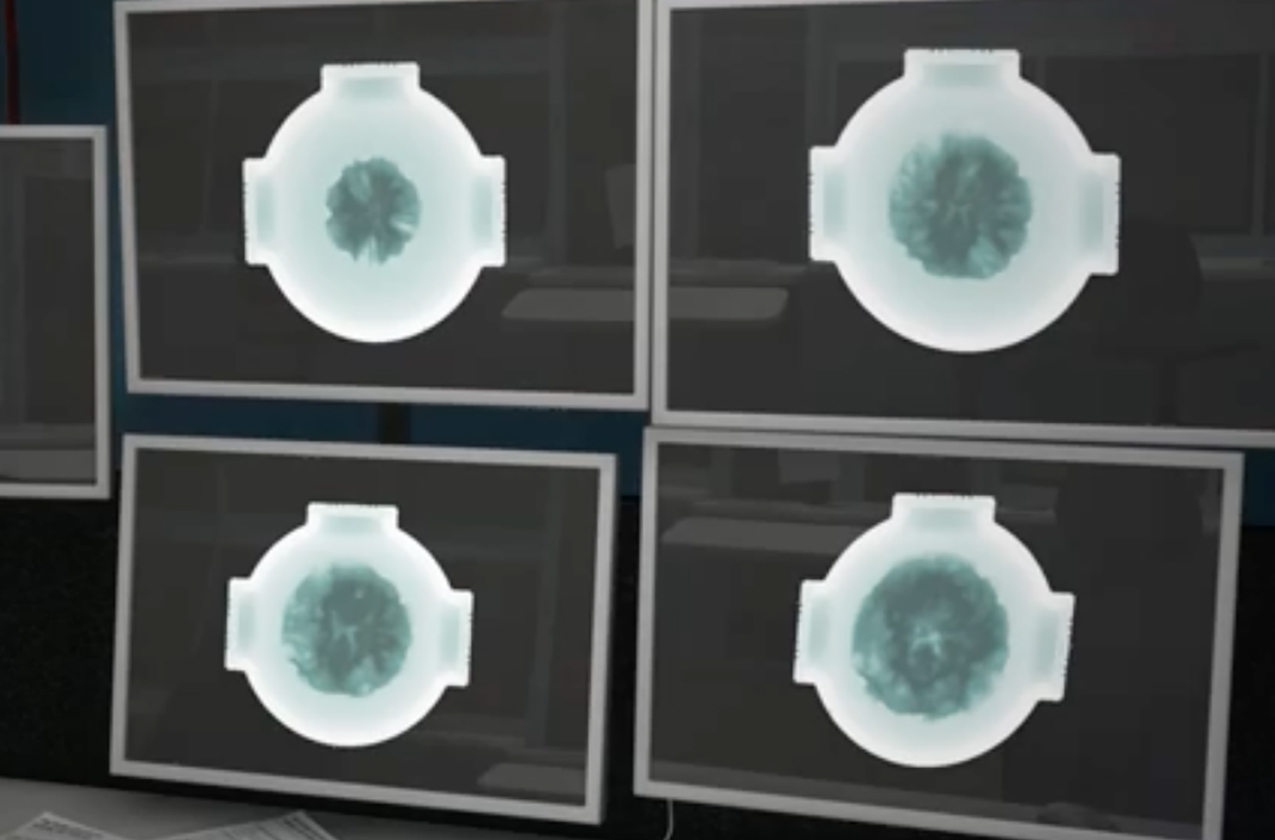
Like LANSCE, DARHT is aging. Axis one entered service in 1999, and axis two was commissioned in 2008; each axis has been “fired” more than 40,000 times for hydrotests and other applications, including for the recent Moonshine experiment, in which the accelerator beam was used to change the state of a small amount of plutonium. Also like LANSCE, DARHT is being called on to support more research than ever. Currently, the Laboratory conducts between six and eight hydrotests per year. However, NNSA expects that 10–15 hydrotests will be needed each year to meet mission objectives. Many non-hydrotests, such as follow up experiments to Moonshine, are also necessary.
To address DARHT’s age and enable it to sustain more tests, upgrades to the facility are needed, says George Laity, who leads the DARHT Capability Expansion Project (DCX). “These integrated weapon experiments are equivalent to modern versions of past underground tests,” Laity says. “They provide the certification basis for stockpile stewardship, so they’re very important tests. And the farther we get from underground testing, the more we need to successfully deliver these experiments.”
The DCX project has three goals. The first has to do with upgrading the facility’s infrastructure—aging parts of the facility, such as the cooling towers, HVAC system, and various concrete structures. The second is to improve the quality of the data captured by DARHT. The project team is exploring several avenues to achieve this goal, including upping the number of radiographs captured at axis two from four to eight, for example, or adding a third axis to the facility.
The third goal is to enable hydrodynamic tests that take place in simulated complex environments. Currently, almost every hydrotest conducted at DARHT has taken place at ambient pressures and temperatures. In practice, however, nuclear weapons must perform under less-than-optimal conditions, oftentimes after being exposed to challenging environments—after sitting inside a B-52’s bomb bay on a hot runway or flying in sub-freezing temperatures, for example. Enabling DARHT to conduct tests that simulate high temperatures or g-forces would help researchers certify weapons’ performance and reliability.
Since DCX’s launch in 2023, the project team has been assembling a comprehensive list of several hundred objectives and readying the highest-priority projects for implementation. These projects include revitalizing DARHT’s control room, refurbishing the infrastructure at the firing point, adding diagnostic capabilities, and more. Taken together, the suite of upgrades planned for DARHT will ensure that the facility remains a key tool in ensuring the nuclear stockpile’s reliability at a time when DARHT is being asked to accomplish more than ever.
“Ten years from now, we’ll be more than 40 years from the last underground test,” Laity says. “The type of experiments that we’ll need to do in the 2030s to meet evolving mission requirements will be different than they were when DARHT was created. We want to ensure that, for the next several decades, DARHT continues to deliver the critical data that’s needed to assess, certify, and qualify the stockpile.”
Scorpius
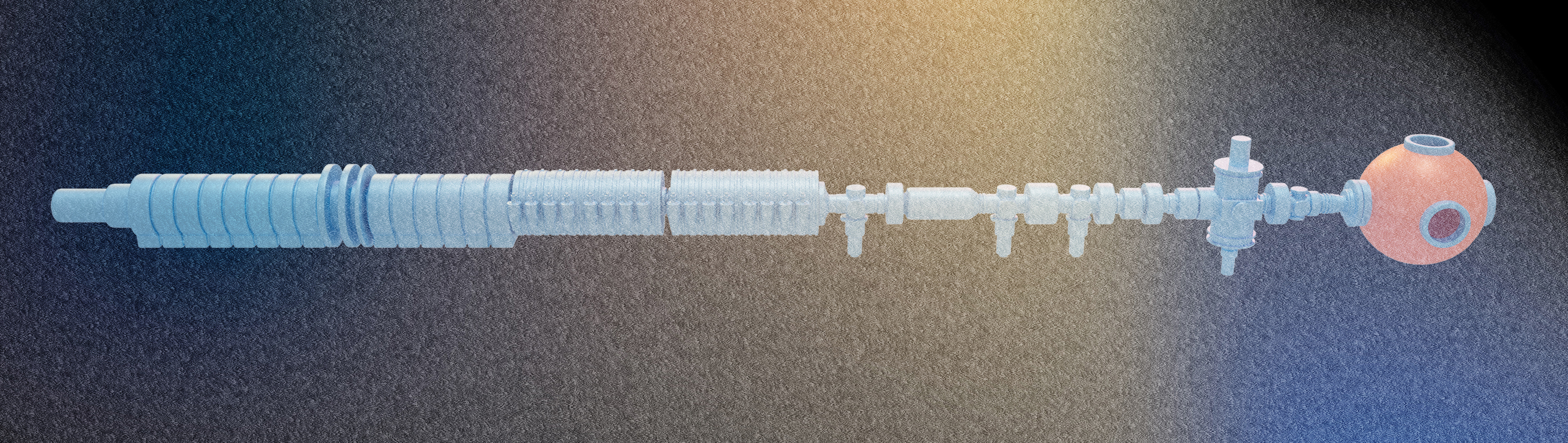
DARHT and Scorpius—the yet-to-be-commissioned accelerator whose development Los Alamos is leading—have a lot in common. Both accelerators are designed to image implosions, and both create high-energy x-rays by bombarding a heavy-metal target with electrons.
Look closer, though, and you’ll find important differences between the two accelerators. The most significant has to do with the fact that unlike DARHT, which uses a surrogate material as a stand-in for plutonium in experiments that model nuclear weapon components, Scorpius will use real plutonium.
The difference between using a surrogate and plutonium in these integrated experiments might seem small. But plutonium is a complicated element, and in the end, only experiments using plutonium will ensure that researchers understand what happens inside a nuclear weapon just before the plutonium goes supercritical, or achieves a self-sustaining chain reaction. (Experiments at Scorpius will approach criticality without crossing that threshold.)
“Scorpius will enable us to close a gap in our understanding of plutonium late in implosions,” says Mike Furlanetto, who directs the Advanced Sources and Detectors project. “This tool will transform our ability to assess and certify the nuclear stockpile.”
DARHT and Scorpius differ in other ways, too. Unlike DARHT, which uses two accelerators to image experiments, Scorpius will use only one. However, advances in accelerator and imaging technologies will allow researchers to gain higher-quality images than was possible when DARHT was commissioned.
One advancement has to do with the “pulses” that Scorpius will use to capture images. DARHT’s second axis, which can capture four separate images of each experimental detonation, works by producing a single, long pulse of electrons that are chopped by a “kicker” into four smaller pulses. Each of these pulses then passes through the experiment to produce an image.
Unfortunately, DARHT’s design doesn’t easily allow for the alteration of the pulses’ length or the amount of time between them, constraining the data that researchers can collect. By contrast, Scorpius will use a solid-state pulsed power source (developed by Lawrence Livermore National Laboratory) and a state-of-the-art electron beam injector (developed by Sandia National Laboratories) that will enable far greater flexibility in imaging. Rather than produce one long pulse that is chopped into four, Scorpius will produce four separate pulses. Researchers will be able to control the length of each of these pulses and the time that separates them.
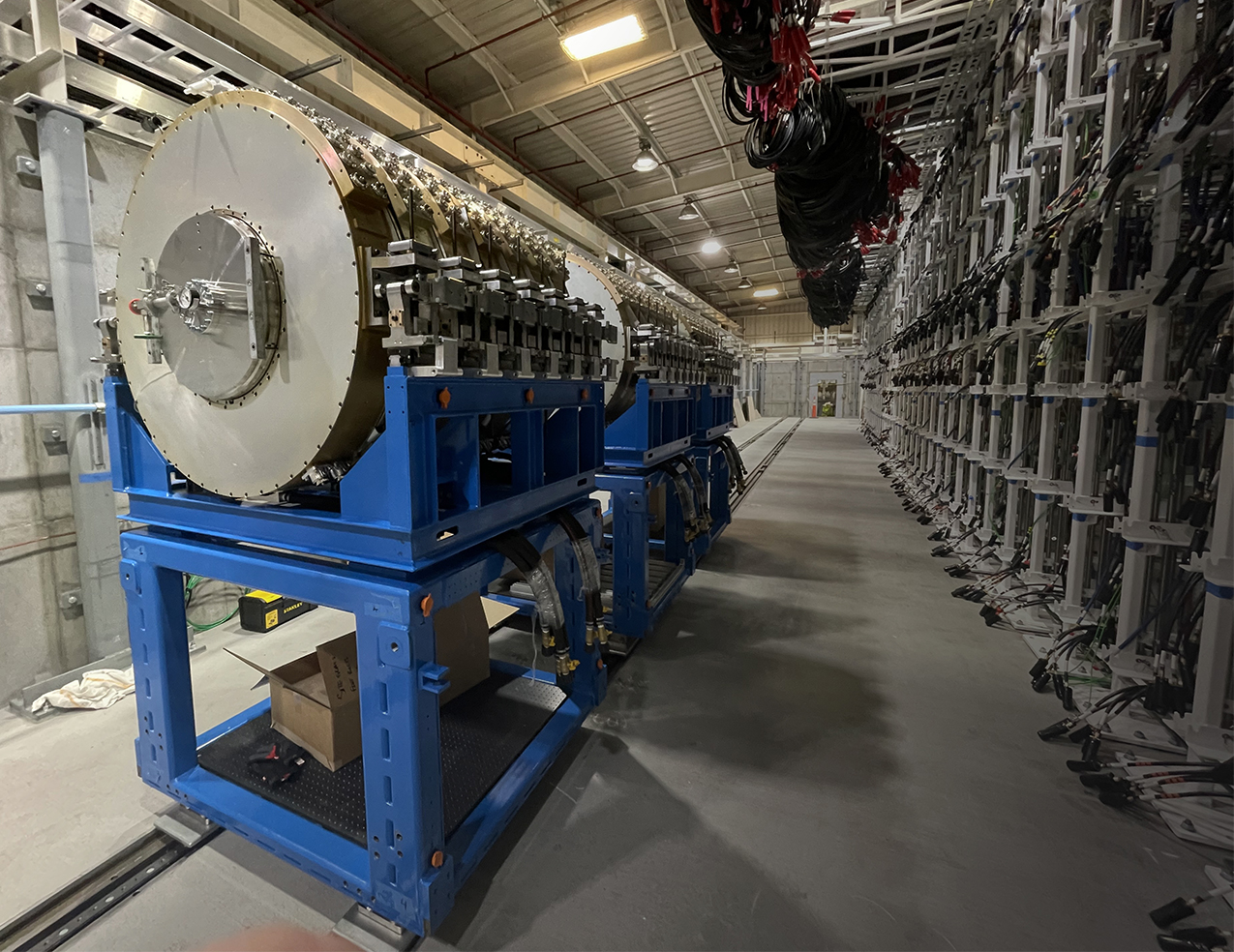
This advance will significantly increase Scorpius’ capabilities. “Rather than design experiments to suit what the accelerator can do, at Scorpius, we’ll be able to tune the pulses to provide what each experiment needs,” Furlanetto says.
Another advancement is in the camera that Scorpius will use to capture images. This camera, which is being developed with the Massachusetts Institute of Technology’s Lincoln Laboratory, will be able to capture images only hundreds of nanoseconds apart. (A nanosecond is one billionth of a second; most commercial digital cameras can capture around 500 images per second, a rate that is several thousand times slower.) One of these cameras will be incorporated into DARHT as well.
Given that both DARHT and Scorpius will be used to conduct integrated tests of mock weapon assemblies, one could wonder what role DARHT will play once Scorpius comes online. Laity says that an upgraded DARHT will remain a vital tool for integrated weapon tests that are focused on non-plutonium weapon assemblies.
“Many of the tests that we need to assess high explosives and other components don’t require special nuclear material,” he says. “The infrastructure that’s being developed for Scorpius will support one or two tests per year. But the number of tests we need to accomplish that don’t require nuclear material is much higher. We’ll still need DARHT to complete those experiments.”
Unlike DARHT and LANSCE, Scorpius won’t be located at Los Alamos, but more than 1,000 feet underground in a tunnel at the Nevada National Security Sites, which is supporting the tri-laboratory collaboration to develop the accelerator. Currently, researchers are building the Integrated Test Stand (ITS) aboveground in North Las Vegas, Nevada. The ITS is a shorter version of Scorpius that allows key components of the accelerator to be tested before they are transported by elevator underground and assembled into the full-scale accelerator. Scorpius itself is expected to enter service in the early 2030s.
An accelerator culture
Whether an accelerator is underground in Nevada or atop a mesa in New Mexico, the Laboratory has the expertise to support its operation. Furlanetto says that Los Alamos is using its experience with accelerators such as LANSCE and DARHT to support the entire nuclear security enterprise and ensure that the nation’s weapons stockpile remains safe, reliable, and effective. “Running accelerators is a culture,” he says. “We at Los Alamos have decades of experience with accelerators, and we are leveraging that experience to meet the national security challenges of the future.” ★